News
Publications
Faculty
System Identification of Nonlinear Aeroelastic Systems
For the past two decades the Volterra series has been successfully utilized for the purpose of flutter prediction, aeroelastic control design and aeroelastic design optimization. The approach has been less successful however, when applied to other important aeroelastic phenomenon such as aerodynamically induced limit cycle oscillations. Similarly to the Taylor series, the Volterra series is a polynomial based approach capable of progressively approximating nonlinear behavior using an infinite summation of quadratic, cubic, and higher-order functional expansions. Unlike the Taylor series however, kernels of the Volterra series are multi-dimensional convolution integrals that are computationally expensive to identify. Thus, even though it is well known that aerodynamic nonlinearities are poorly approximated by quadratic Volterra series models, cubic and higher-order Volterra series truncations can not be identified because their identification costs are too high. We explore a novel, sparse representation of the Volterra series whose identification costs are significantly lower than the identification costs of the full Volterra series. We demonstrate that sparse Volterra reduced-order models are capable of efficiently modeling aerodynamically induced limit cycle oscillations of the prototypical NACA 0012 benchmark model. These results demonstrate for the first time that Volterra series models are capable of modeling aerodynamically induced limit cycle oscillations.

Figure 1. NACA0012 benchmark model flutter boundary.

Figure 2. NACA0012 benchmark model LCO amplitude.
Rotary Wing Aerodynamics
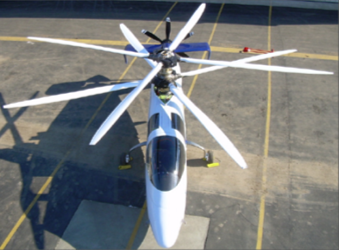
Helicopter are extremely versatile vehicles that have many unique capabilities unavailable to fixed wing aircraft. However, helicopters are inefficient in forward flight relative to a fixed wing aircraft. The fastest production helicopter, the Westland Lynx, holds the helicopter speed record of 216 knots, or about 250 mph, while commercial airliners regularly cruise efficiently at velocities over 600 mph. One of the challenges to efficient forward flight in helicopters is the rapid rise in induced power at high speed, in part due to the requirement to maintain roll trim. Previous studies have shown that compound helicopters, such as the coaxial rotor used on the X2 technology demonstrator or the wing-rotor system used on the Cheyenne helicopter, offer large power reductions relative to conventional single rotor helicopters.
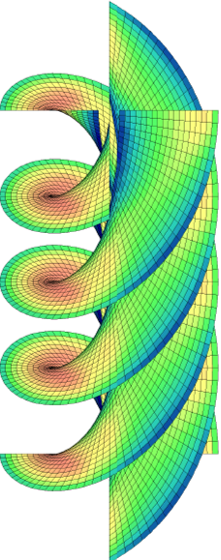
We are researching the optimal aerodynamic rotor design for conventional and compound helicopters. This includes determining the blade twist, chord, and root inputs that produce the most efficient flight. We are also exploring the benefits of higher harmonic blade root control for power reduction. Conventional swashplate control features 1/rev cyclic pitch variation. Past research has shown that higher harmonic control (2/rev, 3/rev, etc.) can provide benefits both in terms of vibration suppression and power reduction. The question we wish to answer is, what aerodynamic advantages are gained with higher harmonic control in compound configurations, and what does the optimal rotor design look like?
We seek to develop computationally efficient methods that can serve as useful tools in the conceptual or preliminary design phases, in addition to gaining insight into the underlying aerodynamics of the helicopter rotor.
Forced response analysis of multi-row compressors
Background
High cycle fatigue failure in turbomachinery is known to be attributed to two major factors, flutter and forced response. Flutter is caused by the blade vibration, which results in negative aerodynamic damping.
This research proposes an effort to investigate computationally the forced response behaviour of a 3.5 stage compressor. This will be in collaboration with Purdue university who will provide us experimental data for the same. This effort is not to just predict responses to compare with experimental data, but will investigate the physics. As an example, forced response computations include three fundamental ingredients: (1) the forcing function, (2) damping (structural and aerodynamic) and (3) mistuned response. As shown in our earlier research, if the errors in the three ingredients cancel out, you can get what appears to be excellent agreement with experimental data.
Tool Development
ANSYS CFX is a time-domain solver widely used in academia and industry. Our research has involved using this tool to conduct multi-row unsteady aerodynamic analyses and expand the knowledge of accurate prediction with affordable computation resource. The analyses have been conducted by using the state-of-the-art interface treatment between stages.
Beyond the pre-defined functionalities, this research helps integrate our understanding in aeromechanics into the usage and develop new features to fulfil the working flow of conducting forced response analysis fully within CFX. Our academic partnership with ANSYS has helped ANSYS add several modules in their software and has led to a mutual benefit for both our research and the expansion of CFX to incorporate specific modules for aeromechanical analysis. The research intends to contribute to the development of existing methods within ANSYS particularly the time transformation (TT) method which has been used to model non-uniform pitch ratio problems. This method when extended to capture multiple frequencies across multiple rows has the capability of reducing a multi stage compressor to a few rows and a few passages per row. This research seeks to determine the maximum extent to which a domain can be reduced while preserving the fidelity of the problem.
Benefits to industry
The overall benefit to industry and academia will be a significantly improved design and development system that will save time and money. This research will focus on explain the physics behind the presence of each row in the compressor and its impact on forcing. Since the research involves carrying out different multi-row simulations at various crossings and loading conditions the research will answer aeromechanical aspects of compressors including the number of rows required to predict the forcing accurately and the nature of interference physical wave reflection off a neighbouring row has on the forcing. One of the traditional methods of multi-row simulations has been to model the entire wheel .This research will provide modelling guidance for reduced passage techniques making use of state of the art interface treatment available in CFX as well as harmonic balance methods available in an in-house code MUSTANG 2.0.
Non-synchronous vibration (NSV) study of a NACA0012
Vortex-Induced Vibration and Frequency Lock-in of an Airfoil at High Angles of Attack
Background

Fluid flow behind bluff bodies and airfoils at high angles of attack undergoes interesting patterns of vortex formation and shedding. These vortices create an unsteady periodic force on the structure, leading to structural vibrations. These vortex-induced vibrations can become dangerous when, under the right circumstances, the vortex shedding frequency “locks-in” with the natural frequency of the structure, leading to excessive fatigue and possibly failure of the structure.
This potential danger makes this phenomenon a great concern in many application areas, including wings, tall buildings, and turbomachinery. Due to the complex nature of these large scale applications, current CFD simulations are not able to efficiently predict in what conditions this lock in phenomenon will occur. The goal is to determine a way to predict these NSV conditions early in the design process.
About this Project
This research seeks to validate the use of our frequency domain, harmonic balance (HB) CFD solver as a potential tool for the prediction of the conditions in which lock-in phenomenon will occur. In this study, the HB CFD solver is applied to the problem of an NACA0012 airfoil in deep stall and the numerical results are compared with experimental data. The HB CFD solver is used to predict the natural shedding frequency of the vortices for a fixed airfoil at a range of high angles of attack and flow speeds, and to identify the lock-in region for an airfoil in forced oscillation.
Experimental tests are conducted in Duke University’s low speed wind tunnel. The natural shedding frequency is measured for a stationary airfoil at various angles of attacks and flow speeds using a hotwire upstream and 20 pressure transduceras along the midspan. These pressure readings are measured for a total of four seconds, sampled around 256 Hz. First, the airfoil will be stationary for baseline measurements. Finally, the airfoil is allowed to oscillate freely within a set range, in order for a lock-in region to develop.
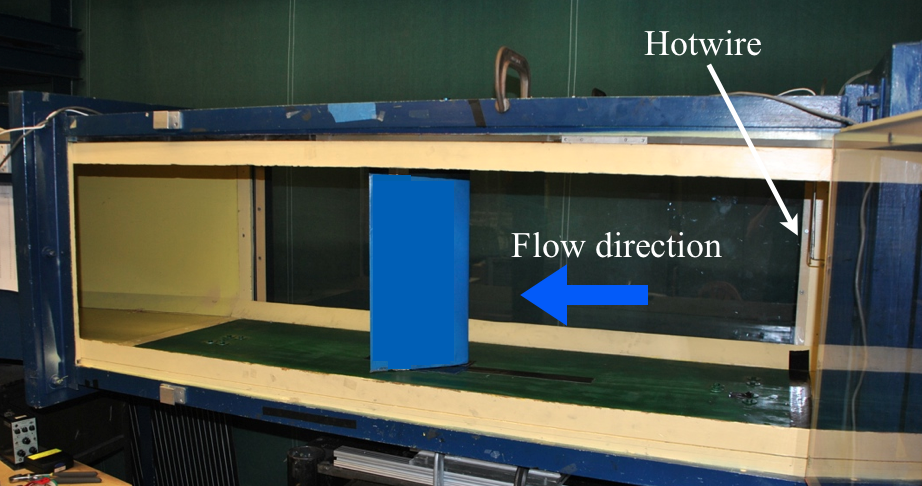
Results and Conclusions
The HB CFD predictions and the experimental data show fairly good agreement for natural shedding frequency measurements of the fixed airfoil. For the oscillating airfoil, the CFD results show a narrower lock-in region than the experimental data, which can be attributed to the chaotic behavior of the vortex patterns on the edges of the lock-in region. Experimentally, further work will be done to precisely identify the lock-in region and allow better comparison with the HB CFD predictions.